Unlock Server Security with Cutting-Edge Cryptography: In today’s interconnected world, server security is paramount. Cyber threats are constantly evolving, demanding sophisticated defenses. This exploration delves into the critical role of modern cryptography in safeguarding your servers from increasingly sophisticated attacks, examining techniques from symmetric and asymmetric encryption to advanced methods like homomorphic encryption and blockchain integration. We’ll cover practical implementation strategies, best practices, and future trends to ensure your data remains protected.
From understanding common vulnerabilities and the devastating impact of data breaches to implementing robust SSL/TLS configurations and secure VPNs, this guide provides a comprehensive overview of how cutting-edge cryptographic techniques can bolster your server’s defenses. We will also explore the crucial aspects of database encryption, secure remote access, and proactive security monitoring, equipping you with the knowledge to build a resilient and secure server infrastructure.
Introduction to Server Security Threats
Server security is paramount in today’s interconnected world, yet maintaining a robust defense against ever-evolving threats remains a significant challenge for organizations of all sizes. The consequences of a successful attack can range from minor service disruptions to catastrophic data loss and reputational damage, highlighting the critical need for proactive security measures and a deep understanding of potential vulnerabilities.The digital landscape is rife with malicious actors constantly seeking exploitable weaknesses in server infrastructure.
These vulnerabilities, if left unpatched or improperly configured, provide entry points for attacks leading to data breaches, system compromise, and denial-of-service disruptions. Understanding these threats and their potential impact is the first step towards building a resilient and secure server environment.
Common Server Vulnerabilities
Several common vulnerabilities are frequently exploited by attackers. These weaknesses often stem from outdated software, misconfigurations, and insufficient security practices. Addressing these vulnerabilities is crucial to mitigating the risk of a successful attack. For example, SQL injection attacks exploit vulnerabilities in database interactions, allowing attackers to manipulate database queries and potentially access sensitive data. Cross-site scripting (XSS) attacks inject malicious scripts into websites, allowing attackers to steal user data or redirect users to malicious sites.
Remote code execution (RCE) vulnerabilities allow attackers to execute arbitrary code on the server, potentially granting them complete control. Finally, insecure network configurations, such as open ports or weak passwords, can significantly increase the risk of unauthorized access.
Impact of Data Breaches on Organizations
Data breaches resulting from server vulnerabilities have far-reaching consequences for organizations. The immediate impact often includes financial losses due to investigation costs, legal fees, regulatory penalties, and remediation efforts. Beyond the direct financial impact, reputational damage can be severe, leading to loss of customer trust and diminished brand value. This can result in decreased sales, difficulty attracting investors, and challenges in recruiting and retaining talent.
Furthermore, data breaches can expose sensitive customer information, leading to identity theft, fraud, and other harms that can have long-lasting consequences for affected individuals. Compliance violations related to data privacy regulations, such as GDPR or CCPA, can result in substantial fines and legal repercussions.
Examples of Real-World Server Security Incidents
Several high-profile server security incidents illustrate the devastating consequences of vulnerabilities. The 2017 Equifax data breach, resulting from an unpatched Apache Struts vulnerability, exposed the personal information of nearly 150 million individuals. This breach resulted in significant financial losses for Equifax, legal settlements, and lasting reputational damage. The 2013 Target data breach, compromising millions of customer credit card numbers, demonstrated the vulnerability of large retail organizations to sophisticated attacks.
This incident highlighted the importance of robust security measures throughout the entire supply chain. These examples underscore the critical need for proactive security measures and continuous monitoring to mitigate the risk of similar incidents.
Understanding Modern Cryptographic Techniques
Modern cryptography is the cornerstone of secure server communication, providing confidentiality, integrity, and authentication. Understanding the underlying principles of various cryptographic techniques is crucial for implementing robust server security measures. This section delves into symmetric and asymmetric encryption algorithms, highlighting their strengths, weaknesses, and applications in securing server infrastructure. The role of digital signatures in verifying server authenticity will also be examined.
Symmetric Encryption Algorithms and Their Applications in Server Security
Symmetric encryption uses a single secret key to both encrypt and decrypt data. This approach is generally faster than asymmetric encryption, making it suitable for encrypting large amounts of data. Common symmetric algorithms include AES (Advanced Encryption Standard) and ChaCha20. AES, particularly in its 256-bit key variant, is widely considered a highly secure algorithm and is frequently employed in securing data at rest and in transit on servers.
ChaCha20, known for its speed and performance on certain hardware architectures, is increasingly used in protocols like TLS 1.3. In server security, symmetric encryption is often used to protect sensitive data stored on the server, encrypting data transmitted between the server and clients, and securing backups. For instance, AES-256 might be used to encrypt database files, while ChaCha20 could be employed in the TLS handshake to establish a secure connection.
Comparison of Symmetric and Asymmetric Encryption Algorithms
Symmetric encryption, while fast, suffers from key distribution challenges: securely sharing the secret key between communicating parties can be difficult. Asymmetric encryption, on the other hand, uses a pair of keys: a public key for encryption and a private key for decryption. The public key can be widely distributed, eliminating the key exchange problem. RSA (Rivest-Shamir-Adleman) and ECC (Elliptic Curve Cryptography) are prominent asymmetric algorithms.
RSA relies on the difficulty of factoring large numbers, while ECC leverages the properties of elliptic curves. ECC generally offers comparable security with shorter key lengths than RSA, making it more efficient for resource-constrained environments. In server security, asymmetric encryption is commonly used for key exchange (e.g., Diffie-Hellman), digital signatures, and encrypting smaller amounts of data where speed is less critical than the security of key management.
Robust server security, achieved through cutting-edge cryptography, is paramount in today’s digital landscape. Protecting sensitive data requires a multi-faceted approach, including strong encryption and secure access controls; understanding how to best serve your customers is also crucial, as detailed in this insightful article on 14 Metode Revolusioner Customer Service Digital 2025. Ultimately, a secure infrastructure bolsters trust, a key element for successful customer interactions and ultimately, a thriving business model dependent on strong server security.
For example, an SSL/TLS handshake might use ECC for key exchange, while the subsequent encrypted communication utilizes a symmetric cipher like AES for efficiency.
Digital Signatures and Server Authentication
Digital signatures provide a mechanism for verifying the authenticity and integrity of data. They utilize asymmetric cryptography. A digital signature is created by hashing the data and then encrypting the hash using the sender’s private key. The recipient can then verify the signature using the sender’s public key. If the verification process is successful, it confirms that the data originated from the claimed sender and has not been tampered with.
In server security, digital signatures are essential for authenticating servers and ensuring the integrity of software updates. For example, a server might use a digital signature to verify the authenticity of a software update downloaded from a repository, preventing malicious code from being installed.
Hypothetical Scenario Illustrating the Use of Digital Signatures for Secure Communication
Imagine a secure online banking system. The bank server holds a private key and publishes its corresponding public key. When a user wants to log in, the server sends the user a challenge (a random number). The user encrypts this challenge using the server’s public key, performs a cryptographic operation (like a hash), and then encrypts the result with their own private key, creating a digital signature.
The user sends this signature back to the server. The server decrypts the signature using the user’s public key (previously obtained during registration) and compares it with the original challenge. If the comparison matches, the server authenticates the user. This ensures that only the legitimate user with access to their private key can successfully log in, preventing unauthorized access. This process utilizes digital signatures to authenticate the user’s request and prevents man-in-the-middle attacks.
Implementing Cutting-Edge Cryptography for Enhanced Security
Modern server security relies heavily on robust cryptographic techniques to protect sensitive data and maintain the integrity of online interactions. Implementing cutting-edge cryptography involves choosing the right algorithms, managing keys effectively, and configuring secure communication protocols. This section details best practices for achieving enhanced server security through the strategic use of modern cryptographic methods.
Elliptic Curve Cryptography (ECC) for Key Exchange
Elliptic curve cryptography offers significant advantages over traditional RSA for key exchange, particularly in resource-constrained environments or where smaller key sizes are desired while maintaining a high level of security. ECC achieves the same level of security as RSA but with significantly shorter key lengths. This translates to faster computation, reduced bandwidth consumption, and improved performance, making it ideal for securing high-traffic servers and mobile applications.
For example, a 256-bit ECC key offers comparable security to a 3072-bit RSA key. This efficiency gain is crucial in scenarios where processing power is limited or bandwidth is a critical constraint. The smaller key sizes also contribute to faster digital signature verification and encryption/decryption processes.
Key Management and Rotation Best Practices
Effective key management is paramount to maintaining the security of any cryptographic system. This involves a robust process for generating, storing, using, and ultimately rotating cryptographic keys. Best practices include using hardware security modules (HSMs) for secure key storage, implementing strong key generation algorithms, and establishing strict access control policies to limit who can access and manage keys.
Regular key rotation, ideally on a predefined schedule (e.g., every 90 days or annually), minimizes the impact of a potential key compromise. Automated key rotation systems can streamline this process and ensure consistent security updates. Furthermore, a well-defined key lifecycle management process, including procedures for key revocation and emergency key recovery, is crucial for comprehensive security.
Configuring SSL/TLS Certificates with Strong Cipher Suites
SSL/TLS certificates are the cornerstone of secure communication over the internet. Proper configuration involves selecting strong cipher suites that offer a balance of security, performance, and compatibility. This typically involves using TLS 1.3 or later, which deprecates weaker protocols and cipher suites. A step-by-step guide for configuring a server with a strong SSL/TLS configuration might involve:
1. Obtain a certificate from a trusted Certificate Authority (CA)
This ensures that clients trust the server’s identity.
2. Install the certificate on the server
This involves configuring the web server (e.g., Apache, Nginx) to use the certificate.
3. Configure strong cipher suites
This requires specifying the preferred cipher suites in the server’s configuration file, prioritizing those using modern algorithms like ChaCha20-Poly1305 or AES-256-GCM.
4. Enable Perfect Forward Secrecy (PFS)
This ensures that even if a long-term key is compromised, past communications remain secure. This typically involves using ephemeral Diffie-Hellman (DHE) or Elliptic Curve Diffie-Hellman (ECDHE) key exchange.
5. Regularly update the certificate
Certificates have an expiration date, and renewing them before expiration is critical to maintain security.
SSL/TLS Protocol Comparison, Unlock Server Security with Cutting-Edge Cryptography
Protocol | Key Exchange | Cipher Suites | Security Features |
---|---|---|---|
TLS 1.0 | Various, including weak options | Many weak and vulnerable options | Basic encryption, vulnerable to various attacks |
TLS 1.1 | Improved over TLS 1.0 | Some improvements, but still vulnerable | Improved encryption, but still vulnerable to attacks |
TLS 1.2 | Stronger options available | More robust cipher suites | Significantly improved security over previous versions, but vulnerable to certain attacks if not configured correctly. |
TLS 1.3 | ECDHE preferred | Modern, high-security cipher suites | Enhanced security, improved performance, and forward secrecy by default. Deprecates weak ciphers and protocols. |
Secure Remote Access and VPNs
VPNs (Virtual Private Networks) are crucial for securing remote access to servers and internal networks. They establish encrypted connections over potentially insecure public networks, protecting sensitive data from eavesdropping and unauthorized access. This section explores how VPNs leverage cryptography, the importance of robust authentication, a comparison of popular VPN protocols, and best practices for secure VPN implementation.
VPNs utilize cryptography to create secure tunnels between a client device and a server. Data transmitted through this tunnel is encrypted, rendering it unreadable to any unauthorized party intercepting the connection. This encryption is typically achieved using symmetric-key cryptography for speed and efficiency, while asymmetric-key cryptography secures the initial handshake and key exchange. The specific algorithms used vary depending on the chosen VPN protocol.
VPN Cryptographic Mechanisms
VPNs employ a combination of encryption and authentication protocols. The encryption process ensures confidentiality, making the transmitted data unintelligible without the correct decryption key. Authentication verifies the identity of both the client and the server, preventing unauthorized access. The process often involves digital certificates and key exchange mechanisms, like Diffie-Hellman, to securely establish a shared secret key used for symmetric encryption.
The strength of the VPN’s security directly depends on the strength of these cryptographic algorithms and the integrity of the implementation.
Strong Authentication Methods for VPN Access
Strong authentication is paramount for secure VPN access. Multi-factor authentication (MFA) is highly recommended, combining something the user knows (password), something the user has (security token), and something the user is (biometric authentication). This layered approach significantly reduces the risk of unauthorized access, even if one factor is compromised. Other robust methods include using strong, unique passwords, regularly updating passwords, and leveraging smart cards or hardware security keys for enhanced security.
Implementing robust password policies and enforcing regular password changes are vital to mitigate risks associated with weak or compromised credentials.
Comparison of VPN Protocols: OpenVPN and WireGuard
OpenVPN and WireGuard are two popular VPN protocols, each with its strengths and weaknesses. OpenVPN, a mature and widely supported protocol, offers a high degree of configurability and flexibility, supporting various encryption algorithms and authentication methods. However, it can be relatively resource-intensive, impacting performance. WireGuard, a newer protocol, is known for its simplicity, speed, and strong security, using modern cryptographic primitives.
While it offers excellent performance, its smaller community and less extensive feature set might be a concern for some users. The choice between these protocols depends on the specific security requirements and performance considerations of the deployment. For instance, resource-constrained environments might favor WireGuard’s efficiency, while organizations needing highly customizable security features might prefer OpenVPN.
Best Practices for Configuring and Maintaining Secure VPN Connections
Implementing and maintaining secure VPN connections requires careful consideration of several factors. The following list Artikels key best practices:
- Use strong encryption algorithms (e.g., ChaCha20-Poly1305 for WireGuard, AES-256-GCM for OpenVPN).
- Employ robust authentication mechanisms (e.g., MFA, certificate-based authentication).
- Regularly update VPN server software and client applications to patch security vulnerabilities.
- Implement strict access control policies, limiting VPN access only to authorized users and devices.
- Monitor VPN logs for suspicious activity and promptly address any security incidents.
- Use a trusted VPN provider with a proven track record of security and privacy.
- Regularly audit and review VPN configurations to ensure they remain secure and effective.
Database Encryption and Data Protection
Protecting sensitive data stored in databases is paramount for any organization. Database encryption, both at rest and in transit, is a crucial component of a robust security strategy. This section explores various techniques, their trade-offs, potential implementation challenges, and practical solutions, focusing on the encryption of sensitive data within databases.Database encryption methods can be broadly categorized into two types: encryption at rest and encryption in transit.
Encryption at rest protects data stored on the database server’s hard drives or storage media, while encryption in transit secures data as it travels between the database server and clients. Choosing the right method often depends on the specific security requirements, performance considerations, and the type of database being used.
Database Encryption at Rest
Encryption at rest involves encrypting data before it’s written to disk. This protects data from unauthorized access even if the server is compromised. Several methods exist, each with its own advantages and disadvantages. Transparent Data Encryption (TDE) is a common approach, managed by the database system itself. It often uses symmetric encryption, where the same key is used for encryption and decryption, with a master key protected separately.
File-system level encryption, on the other hand, encrypts the entire database file, offering a simpler implementation but potentially impacting performance more significantly. Columnar encryption provides granular control, encrypting only specific columns containing sensitive information, improving performance compared to full-table encryption.
Database Encryption in Transit
Encryption in transit protects data as it travels between the database server and applications or clients. This is typically achieved using Transport Layer Security (TLS) or Secure Sockets Layer (SSL), which establishes an encrypted connection. All communication is encrypted, protecting data from eavesdropping or man-in-the-middle attacks. The implementation is generally handled at the network level, requiring configuration of the database server and client applications to use secure protocols.
Trade-offs Between Database Encryption Methods
The choice of encryption method involves several trade-offs. TDE offers ease of use and centralized management but might slightly impact performance. File-system level encryption is simpler to implement but can be less granular and affect performance more noticeably. Columnar encryption offers a balance, allowing for granular control and potentially better performance than full-table encryption, but requires more complex configuration and management.
Finally, encryption in transit, while crucial for securing data in motion, adds a layer of complexity to the network configuration. The optimal choice depends on the specific needs and priorities of the organization, including the sensitivity of the data, performance requirements, and available resources.
Challenges in Implementing Database Encryption and Solutions
Implementing database encryption can present several challenges. Key management is crucial; securely storing and managing encryption keys is paramount to prevent data breaches. Performance overhead is another concern; encryption and decryption operations can impact database performance. Integration with existing applications might require modifications to support encrypted connections or data formats. Finally, compliance requirements need careful consideration; organizations must comply with relevant regulations and standards related to data security and privacy.
Solutions include robust key management systems, optimizing encryption algorithms for performance, careful planning during application integration, and adherence to relevant industry best practices and regulatory frameworks.
Encrypting Sensitive Data with OpenSSL
OpenSSL is a powerful, open-source cryptographic library that can be used to encrypt and decrypt data. While OpenSSL itself doesn’t directly encrypt entire databases, it can be used to encrypt sensitive data within applications interacting with the database. For example, before inserting sensitive data into a database, an application can use OpenSSL to encrypt the data using a strong symmetric encryption algorithm like AES- The encrypted data is then stored in the database, and the application can decrypt it using the same key when retrieving it.
This requires careful key management and secure storage of the encryption key. The specific implementation would depend on the programming language and database system being used, but the core principle remains the same: using OpenSSL to encrypt sensitive data before it enters the database and decrypting it upon retrieval. Consider the example of encrypting a password before storing it in a user table.
The application would use OpenSSL’s AES-256 encryption to encrypt the password with a randomly generated key, store both the encrypted password and the key (itself encrypted with a master key) in the database. Upon authentication, the application would retrieve the key, decrypt it using the master key, and then use it to decrypt the password before comparison. This example demonstrates a practical application of OpenSSL for database security, although it’s crucial to remember that this is a simplified illustration and real-world implementations require more sophisticated techniques for key management and security.
Advanced Cryptographic Techniques for Server Protection: Unlock Server Security With Cutting-Edge Cryptography
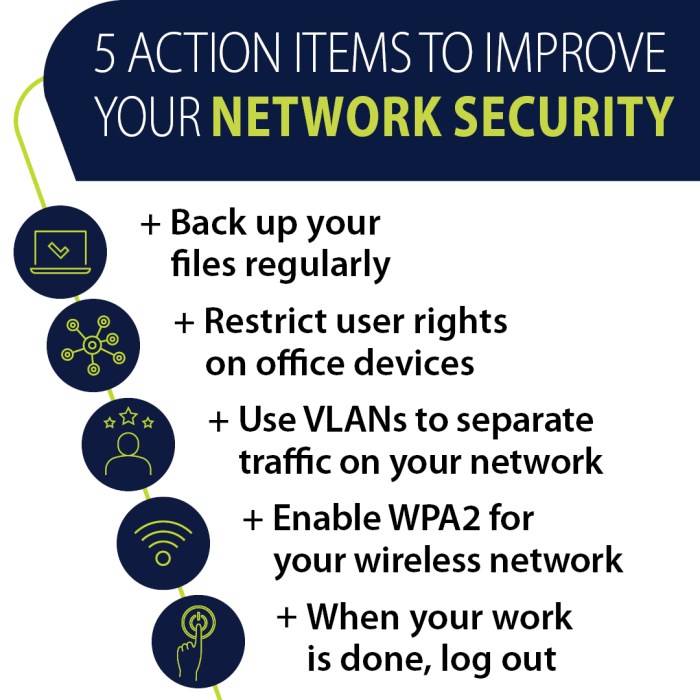
Modern server security demands more than traditional encryption methods. The increasing sophistication of cyber threats necessitates the adoption of advanced cryptographic techniques to ensure data confidentiality, integrity, and availability. This section explores several cutting-edge approaches that significantly enhance server protection.
Homomorphic Encryption and Secure Cloud Computing
Homomorphic encryption allows computations to be performed on encrypted data without decryption. This groundbreaking technology enables secure cloud computing by permitting processing of sensitive information without ever revealing its plaintext form to the cloud provider. For example, a financial institution could outsource complex data analysis to a cloud service, maintaining the confidentiality of client data throughout the process. The cloud provider can perform calculations on the encrypted data, returning the encrypted result, which can then be decrypted by the institution with the private key.
This eliminates the risk of data breaches during cloud storage and processing. Different types of homomorphic encryption exist, with fully homomorphic encryption (FHE) offering the most comprehensive capabilities, although it comes with significant computational overhead. Partially homomorphic encryption schemes offer a balance between functionality and performance.
Blockchain Technology’s Role in Server Security
Blockchain’s distributed ledger technology can significantly enhance server security. Its immutable record-keeping capabilities provide an auditable trail of all server activities, making it difficult to tamper with system logs or data. This enhanced transparency improves accountability and strengthens security posture. Furthermore, blockchain can be used for secure access control, enabling decentralized identity management and authorization. Imagine a scenario where access to a server is granted only when a specific cryptographic key, held by multiple authorized parties, is combined through blockchain consensus.
This multi-signature approach reduces the risk of unauthorized access, even if one key is compromised.
Zero-Knowledge Proofs for Secure Authentication
Zero-knowledge proofs allow users to prove their identity or knowledge of a secret without revealing the secret itself. This is crucial for server authentication and access control, minimizing the risk of exposing sensitive credentials. For example, a user can prove they possess a specific private key without revealing the key’s value. This is achieved through cryptographic protocols that verify the possession of the key without exposing its content.
This technique safeguards against credential theft and strengthens the overall security of the authentication process. Practical applications include secure login systems and verifiable credentials, significantly reducing the vulnerability of traditional password-based systems.
Emerging Cryptographic Trends in Server Security
The landscape of cryptography is constantly evolving. Several emerging trends are poised to further enhance server security:
- Post-Quantum Cryptography: The development of quantum computers threatens the security of current cryptographic algorithms. Post-quantum cryptography aims to develop algorithms resistant to attacks from quantum computers.
- Differential Privacy: This technique adds carefully designed noise to data to protect individual privacy while still enabling meaningful statistical analysis. It’s particularly useful in scenarios involving sensitive user data.
- Multi-Party Computation (MPC): MPC allows multiple parties to jointly compute a function over their private inputs without revealing anything beyond the output. This is valuable for collaborative data processing while preserving data confidentiality.
- Hardware-Based Security Modules (HSMs): HSMs provide a secure environment for cryptographic operations, protecting sensitive keys and cryptographic algorithms from external attacks.
- Lattice-Based Cryptography: Lattice-based cryptography is considered a promising candidate for post-quantum cryptography due to its perceived resistance to attacks from both classical and quantum computers.
Monitoring and Auditing Server Security
Proactive monitoring and regular security audits are crucial for maintaining the integrity and confidentiality of server systems. Neglecting these practices significantly increases the risk of breaches, data loss, and financial repercussions. A robust security posture requires a multi-layered approach, encompassing both preventative measures (like strong cryptography) and reactive mechanisms for detecting and responding to threats.Regular security audits and penetration testing identify vulnerabilities before malicious actors can exploit them.
This proactive approach allows for timely remediation, minimizing the impact of potential breaches. Effective log monitoring provides real-time visibility into server activity, enabling swift detection of suspicious behavior. A well-designed incident response system ensures efficient containment and recovery in the event of a security incident.
Regular Security Audits and Penetration Testing
Regular security audits involve systematic evaluations of server configurations, software, and network infrastructure to identify weaknesses. Penetration testing simulates real-world attacks to assess the effectiveness of security controls. These combined approaches provide a comprehensive understanding of the server’s security posture. Audits should be conducted at least annually, with more frequent assessments for critical systems. Penetration testing should be performed at least semi-annually, employing both black-box (attacker has no prior knowledge) and white-box (attacker has some prior knowledge) testing methodologies to gain a complete picture of vulnerabilities.
For example, a recent audit of a financial institution’s servers revealed a critical vulnerability in their web application firewall, which was promptly patched after the audit.
Monitoring Server Logs for Suspicious Activity
Server logs contain valuable information about system activity, including user logins, file access, and network connections. Regularly reviewing these logs for anomalies is essential for early threat detection. Key indicators of compromise (KIOCs) include unusual login attempts from unfamiliar locations, excessive file access requests, and unusual network traffic patterns. Effective log monitoring involves using centralized log management tools that aggregate logs from multiple servers and provide real-time alerts for suspicious activity.
For instance, a sudden spike in failed login attempts from a specific IP address could indicate a brute-force attack.
System for Detecting and Responding to Security Incidents
A well-defined incident response plan is critical for minimizing the impact of security breaches. This plan should Artikel procedures for identifying, containing, eradicating, recovering from, and learning from security incidents. It should include clearly defined roles and responsibilities, communication protocols, and escalation paths. The plan should also detail procedures for evidence collection and forensic analysis. Regular drills and simulations help ensure the plan’s effectiveness and team preparedness.
A hypothetical scenario: a ransomware attack encrypts critical data. The incident response plan would dictate the steps to isolate the affected systems, restore data from backups, and investigate the attack’s origin.
Security Information and Event Management (SIEM) Tools
SIEM tools consolidate security logs from various sources, providing a centralized view of security events. They employ advanced analytics to detect patterns and anomalies, alerting security personnel to potential threats. Examples include Splunk, IBM QRadar, and LogRhythm. Splunk, for example, offers real-time log monitoring, threat detection, and incident response capabilities. QRadar provides advanced analytics and threat intelligence integration.
LogRhythm offers automated incident response workflows and compliance reporting. The choice of SIEM tool depends on the organization’s specific needs and budget.
Illustrative Examples of Secure Server Architectures
Designing a truly secure server architecture requires a layered approach, combining multiple security mechanisms to create a robust defense against a wide range of threats. This involves careful consideration of network security, application security, and data security, all underpinned by strong cryptographic practices. A well-designed architecture minimizes the impact of successful attacks and ensures business continuity.A robust server architecture typically incorporates firewalls to control network access, intrusion detection systems (IDS) to monitor network traffic for malicious activity, and encryption to protect data both in transit and at rest.
These elements work in concert to provide a multi-layered defense. The specific implementation will vary depending on the organization’s needs and risk tolerance, but the core principles remain consistent.
Secure Server Architecture Example: A Layered Approach
This example illustrates a secure server architecture using a combination of firewalls, intrusion detection systems, and cryptography. The architecture is designed to protect a web server handling sensitive customer data.
Visual Representation (Text-Based):
Imagine a layered diagram. At the outermost layer is a Firewall, acting as the first line of defense. It filters incoming and outgoing network traffic based on predefined rules, blocking unauthorized access attempts. Inside the firewall is a Demilitarized Zone (DMZ) hosting the web server. The DMZ provides an extra layer of security by isolating the web server from the internal network.
The web server itself is configured with robust Web Application Firewall (WAF) rules to mitigate application-level attacks like SQL injection and cross-site scripting (XSS). The web server utilizes HTTPS, encrypting all communication between the server and clients using TLS/SSL certificates. An Intrusion Detection System (IDS) monitors network traffic within the DMZ and the internal network, alerting administrators to suspicious activity.
The database server, residing within the internal network, is protected by a separate firewall and employs database-level encryption to protect sensitive data at rest. All communication between the web server and the database server is encrypted using secure protocols. Finally, regular security audits and penetration testing are performed to identify and address vulnerabilities.
Detailed Description: The firewall acts as a gatekeeper, only allowing authorized traffic to pass. The DMZ further isolates the web server, preventing direct access from the internet to the internal network. The WAF protects against application-level attacks. HTTPS encrypts data in transit, protecting it from eavesdropping. The IDS monitors network traffic for malicious activity, providing early warning of potential attacks.
Database-level encryption protects data at rest, preventing unauthorized access even if the database server is compromised. Regular security audits and penetration testing identify and address vulnerabilities before they can be exploited.
Final Conclusion
Securing your servers against modern threats requires a proactive and multi-layered approach. By implementing the cutting-edge cryptographic techniques discussed, coupled with robust security monitoring and regular audits, you can significantly reduce your vulnerability to attacks. This journey into the world of server security highlights the importance of staying ahead of the curve, adopting best practices, and continuously adapting your security strategy to the ever-evolving landscape of cyber threats.
Investing in robust security is not just a cost; it’s an investment in the protection of your valuable data and the continuity of your operations.
Common Queries
What are the key differences between symmetric and asymmetric encryption?
Symmetric encryption uses the same key for both encryption and decryption, offering speed but requiring secure key exchange. Asymmetric encryption uses separate public and private keys, enabling secure key exchange but being slower.
How often should SSL/TLS certificates be rotated?
The frequency depends on the certificate type and risk tolerance, but generally, it’s recommended to rotate certificates at least annually, or more frequently for high-security applications.
What are some common signs of a compromised server?
Unusual network traffic, slow performance, unauthorized access attempts, and unusual log entries are all potential indicators of a compromised server.
How can I choose the right VPN protocol for my needs?
Consider security, performance, and ease of configuration. OpenVPN offers strong security but can be resource-intensive; WireGuard is faster and simpler but might have fewer features.